Metal casting is an essential manufacturing process used to create parts and components for industries ranging from aerospace and automotive to construction and electronics. The success of a casting project depends on many factors, and the design of the metal casting itself is one of the most critical elements. Whether you're working with traditional sand casting, investment casting, or die casting, understanding the key considerations in metal casting design will help ensure your parts are functional, durable, and cost-effective. In this blog, we’ll explore the various factors you need to keep in mind when designing a metal casting, from material selection to the casting process itself.
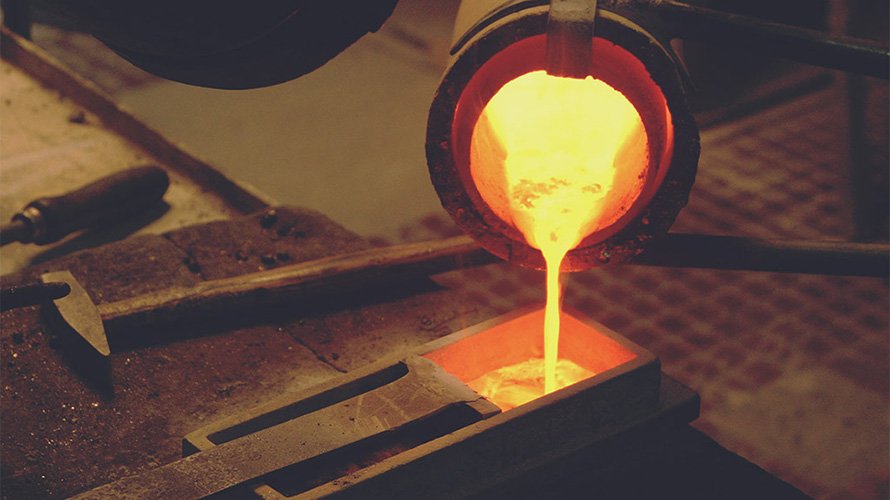
Material Selection: The Foundation of Design
The first step in designing a metal casting is selecting the right material for the job. Different metal alloys offer unique properties such as strength, corrosion resistance, thermal stability, and machinability, which are essential for the final part's performance. Common metals used in casting include aluminum, iron, steel, brass, and bronze, each with its own set of benefits.
· Aluminum Castings: Lightweight, corrosion-resistant, and easy to machine, making it suitable for automotive and aerospace applications.
· Cast Iron: Strong and durable, cast iron is ideal for heavy-duty applications, such as engine blocks or machinery parts.
· Steel Castings: Steel offers high strength and toughness, making it ideal for industrial applications that require heavy-duty performance.
· Brass and Bronze: These copper alloys provide excellent corrosion resistance and are often used for decorative and marine applications.
Properties to Consider in Material Selection
When selecting the right material, you should consider the material's strength, thermal conductivity, corrosion resistance, weight, and the environment in which the part will operate. The choice of material will affect how the casting behaves during the manufacturing process, its final properties, and its cost.
· Strength and Durability: Does the part need to withstand high stress or mechanical forces?
· Corrosion Resistance: Will the part be exposed to moisture, chemicals, or harsh environments?
· Thermal Conductivity: Is the part exposed to extreme temperatures?
· Machinability: How easy will it be to finish and machine the casting post-production?
Design for Casting: Optimizing for the Process
One of the most important considerations in metal casting design is ensuring that the part has consistent wall thickness. If the wall thickness is too thin, the casting may not solidify correctly, leading to defects like shrinkage or porosity. Conversely, excessively thick sections can lead to problems with cooling rates, causing internal stresses, cracks, or warping.
· Uniformity in Wall Thickness: To prevent defects like hot tears and misruns, design the part with even wall thickness across the entire casting.
· Gradual Transitions: Sharp changes in wall thickness can cause stress concentrations and defects. Gradual transitions between sections of varying thickness are ideal.
Ribs and Supports
In some castings, particularly those with complex geometries, the inclusion of ribs or supports can add strength and rigidity to the part. Ribs are often used in structural components like frames, housings, and brackets to improve mechanical properties without significantly increasing weight. However, the size, shape, and placement of these ribs must be carefully considered.
· Rib Thickness: Keep ribs thinner than the main walls to ensure they don’t impede proper cooling or solidification.
· Avoiding Sharp Corners: Sharp corners or angles can create stress points and casting defects. It’s better to design with radii or fillets at transitions.
Draft Angles for Mold Release
Another critical design consideration is the addition of draft angles. A draft angle is a slight taper added to the walls of the casting, which makes it easier to remove the casting from the mold after it has cooled. Without adequate draft, the casting may get stuck in the mold, leading to defects or even mold damage. The optimal draft angle typically ranges from 1° to 5°, depending on the size and complexity of the part.
Designing for Manufacturing Efficiency
While metal casting allows for the creation of complex geometries, it’s still important to design with manufacturing efficiency in mind. Complex shapes and features can increase the cost of tooling, mold-making, and machining, so it’s best to simplify the design where possible.
· Minimize Undercuts: Undercuts—features that prevent the mold from easily separating—can complicate mold design and increase costs. If possible, design the part with minimal or no undercuts.
· Avoid Excessive Detail: Fine details can be challenging to cast accurately, especially in large or heavy parts. Consider how much detail is truly needed in the final product and balance it with the cost and complexity of production.
Optimizing for Material Usage
Casting design should also take material efficiency into account. Wastage during the casting process, such as excessive scrap or overuse of material, can significantly impact both cost and environmental sustainability. Efficient design minimizes the amount of metal used, reduces scrap, and lowers overall production costs.
· Minimize Risers and Sprues: Risers and sprues are channels through which the molten metal flows into the mold. While necessary for some types of casting, they should be minimized to reduce material waste.
· Utilize Simulations: Modern casting simulation software allows designers to predict material flow, cooling rates, and potential defects before physical production begins. Using simulations to optimize material usage is an excellent way to streamline design.
Designing for Post-Processing
After a metal casting is produced, it often requires additional steps like cleaning, machining, or surface treatment. When designing a casting, you should consider how easy it will be to carry out these post-processing tasks. Designing features that are easy to access and machine can help reduce the overall time and cost of the project.
· Machining Allowances: If the part will require machining after casting, leave enough material around critical areas to ensure they can be accurately shaped without removing too much metal.
· Surface Finish: Consider how the surface finish will be achieved. Some castings may need a smoother surface for cosmetic reasons, while others might require a rougher finish for functional purposes, such as better friction characteristics.
Considerations for Casting Defects and Quality Control
Designing a part that minimizes the risk of defects is essential for ensuring the quality and performance of the final product. Common casting defects include:
· Porosity: Small holes or voids within the casting caused by trapped gases. This can be minimized by controlling the cooling rate and ensuring proper venting in the mold.
· Shrinkage: The reduction in volume as the molten metal cools and solidifies. Design features like risers and feeders can help compensate for this by providing extra metal during solidification.
· Cold Shuts: Occur when two streams of molten metal fail to fuse properly during pouring. Cold shuts can be minimized by optimizing gating systems and material flow.
Quality Control Measures in Design
To avoid defects and ensure a high-quality casting, it's important to design with quality control in mind. Implementing design features that facilitate quality checks during the casting process—such as location markings for visual inspection or material flow indicators—can make a big difference in the overall reliability of the part.
· Mold Venting: Proper mold venting allows gases to escape during casting, which reduces the likelihood of defects like porosity.
· Simulation Tools: Using casting simulation software can help identify potential problems, such as areas with slow cooling or poor metal flow, before physical production begins.
Testing and Validation
After a part is cast, various tests may be required to ensure it meets the necessary specifications. These tests could include mechanical property testing (tensile strength, hardness, etc.), dimensional verification, and non-destructive testing (e.g., X-ray inspection). Designing with these tests in mind can make it easier to validate the part’s quality and functionality.
Conclusion
Designing for metal casting involves much more than simply creating a part that looks good. It requires a deep understanding of the material properties, casting process, and manufacturing techniques that affect the performance and cost of the final product. By considering key factors such as material selection, wall thickness, rib design, and post-processing, manufacturers can produce high-quality, cost-effective castings that meet the specific needs of their industries. Whether you're designing a simple bracket or a complex aerospace component, keeping these considerations in mind will lead to better results and a more efficient manufacturing process.